【導(dǎo)讀】薄膜熱電的冷卻器中有幾個(gè)關(guān)鍵優(yōu)點(diǎn),特別適合用于PCR。這些優(yōu)勢包括:較小的尺寸和厚度相同的熱量抽水能力;更快速的熱響應(yīng)(最高10X更大);先進(jìn)的集成能力。同時(shí)小尺寸,能夠使集成到系統(tǒng)的影響最小。
Background
Polymerase chain reaction (PCR) is a scientific technique that amplifies a single or a few copies of a specific piece of DNA by several orders of magnitude, generating thousands to millions of copies of a particular DNA sequence. Developed in 1983, PCR has rapidly become one of the most widely used techniques in molecular biology and for good reason: it is a rapid, inexpensive and simple means of producing relatively large quantities of whole or fractional DNA strands copied from minute quantities of source DNA material even when the source DNA is of relatively poor quality. Applications for PCR include DNA cloning for sequencing, DNA-based phylogeny, or functional analysis of genes; the diagnosis of hereditary diseases; the identification of genetic fingerprints (used in forensic sciences and paternity testing); and the detection and diagnosis of infectious diseases.
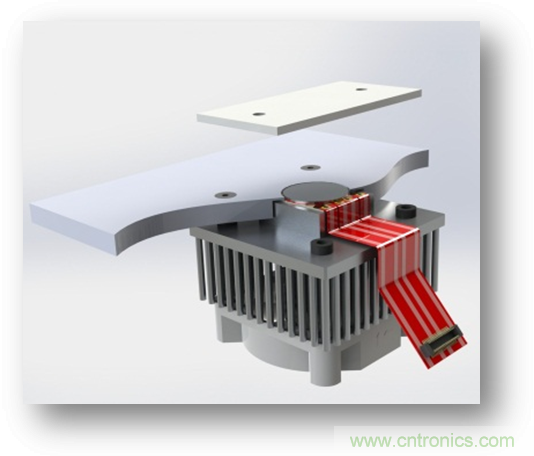
Figure 1: Use of Thin-Film Thermoelectrics in PCR Thermal Cycling
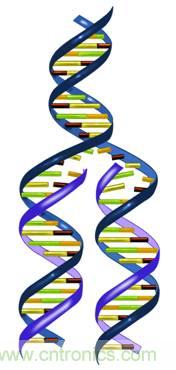
[page]
Market Drivers
Smaller, faster, cheaper is the rallying cry for virtually every successful product on the market, and the PCR market is no exception. It is clear that the PCR market has and will continue to have significant near or above double-digit growth year after year. One of the significant trends in the PCR market today is a movement towards smaller, individually addressable wells (containing the DNA). Historically, systems were comprised of large 96-well thermal cyclers for large industry applications.
Companies are now developing applications that require individually addressable wells, where only a few samples are tested at a time. An additional requirement would be that each well be individually programmable. The requirement to have individually addressable wells enables different reagents to be simultaneously applied to identical DNA samples with different outcomes intended.
Another rapidly developing market is food safety and security. According to a recently published report by Strategic Consulting Inc. (SCI), the market for microbiological testing in the food sector has been growing at nearly 9% every year since 1998. The food sector now accounts for nearly half of the total industrial microbiology market. The SCI report estimates that by 2013, the number of tests carried out worldwide will be nearly 970 million, up from 740 million in 2008. The drivers for this growth are identified as an overall increase in food production, food safety concerns, demands from retailers and an increase in regulatory requirements.
Thermal Cycling and the PCR Process
The PCR process is very versatile. Many types of samples can be analyzed for nucleic acids. Most PCR uses DNA as a target, rather than RNA, because of the stability of the DNA molecule and the ease with which DNA can be isolated. By following a few basic rules, problems can be avoided in the preparation of DNA for the PCR. The essential criteria for any DNA sample is that it contains at least one intact DNA strand encompassing the region to be amplified, and that any impurities are sufficiently diluted so as not to inhibit the polymerization step of the PCR reaction.
PCR requires thermal cycling, i.e., alternately heating and cooling the DNA sample in a defined series of temperature steps. These thermal cycling steps are necessary first to physically separate the two strands in a DNA double helix at a high temperature in a process called DNA melting. At a lower temperature, each strand is then used as the template in DNA synthesis by the DNA polymerase to selectively amplify the target DNA. As the PCR process progresses, the DNA generated is used as a template for replication, setting in motion a chain reaction in which the DNA template is exponentially amplified. Table 1 describes the thermal cycling steps, temperatures, and hold times required in a typical PCR process. The selectivity of PCR results from the use of primers that are complementary to the DNA region targeted for amplification under specific thermal cycling conditions.
A thermal cycler is an automated instrument specifically designed to generate the requisite thermal cycles for PCR. A typical device consists of a metal block with holes where plastic vials holding the PCR reaction mixtures are inserted. The instrument has an integrated heating/cooling unit that is used to systematically raise and lower the temperature of the block. Thermoelectric coolers (TEC) are used for a large number of these systems.
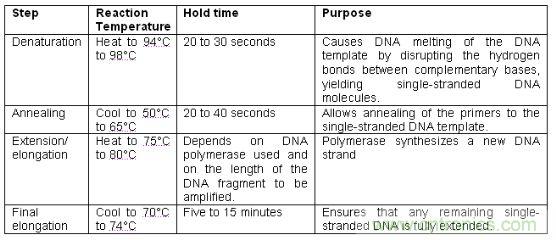
Thermoelectric cooling makes use of the Peltier effect to create a heat flux between the junctions of two different types of materials. A Peltier cooler, heater, or thermoelectric heat pump is a solid-state heat pump that transfers heat from one side of the device to the other side against a temperature gradient (from cold to hot). To do this, electrical energy is required. The electrical energy used for the pumping is converted into additional heat that must be removed from the system (much like the heat from a home air-conditioner unit). A device that operates in this manner may also be called a Peltier device, Peltier diode, Peltier heat pump, solid state refrigerator, or thermoelectric cooler.
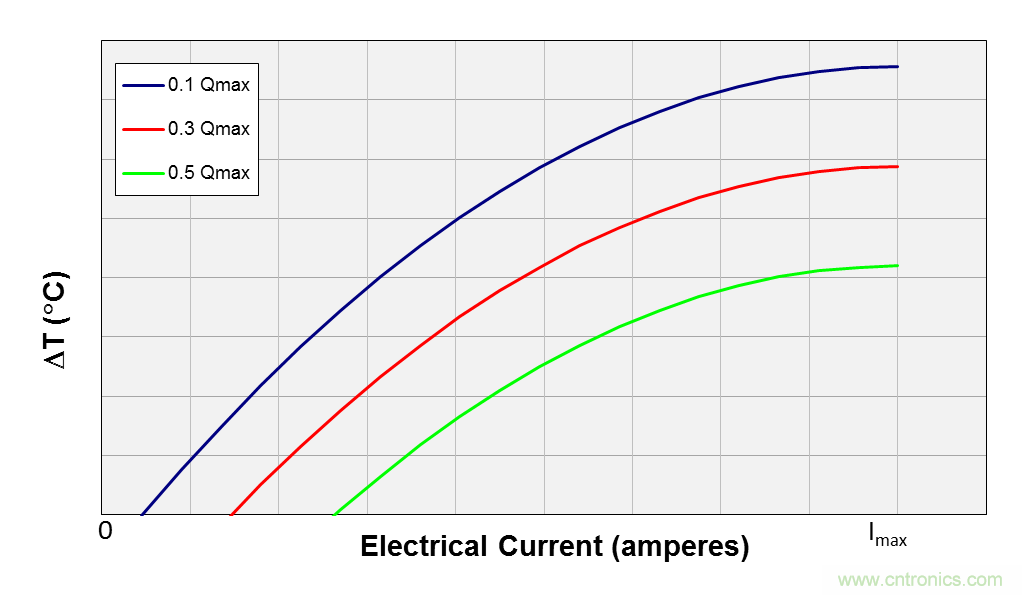
The most basic representation of a thermoelectric cooling device is a cooling curve (Figure 3). The cooling curve represents the ΔT (or temperature difference between the cold and hot sides of the TEC) as a function of the input electrical current to the TEC. There is a different curve for each unique amount of heat being pumped. Figure 2 shows three different examples. As the heat pumped (Q) increases, the amount the TEC can cool is reduced. For all cases, the maximum drive current for the module, Imax, provides the most cooling achievable from that TEC under the given load conditions.
Thin-film thermoelectric coolers (eTECs) operate in the same manner as conventional ones but offer several key advantages that are particularly well suited for PCR. These advantages are:
Smaller footprint and thickness for the same heat pumping capacity (Fig. 4).
More rapid thermal response (up to 10X greater)
Advanced integration capability. The small size enables integration into systems with minimal impact.
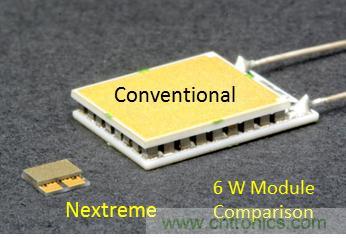
[page]
Thermoelectrics for the PCR Process
The market for PCR is changing. New applications pushing for portability and reduced analysis time offer the promise of large new markets. In order to meet these requirements, systems having smaller lighter quicker TECs are required. Due to their low mass, thin-film thermoelectric devices are particularly well suited for producing rapid temperature changes while also achieving a uniform temperature distribution throughout the block, or enabling different temperatures in different parts of the block. This is particularly useful when testing suitable annealing temperatures for primers, which are required for initiation of the DNA replication sequence.
Thermal cyclers with thin-film technology inside can offer significantly shorter throughput times for DNA amplification. Precision temperature control takes the guesswork out of the traditional "trial and error" methods used in DNA amplification and reduces the need to run multiple trials to get the desired results. Thin-film technology can enable a new generation of thermal cyclers for equipment manufacturers that lower barrier-to-entry and increase opportunities for differentiation.
In 2008, Nextreme successfully demonstrated short cycle time temperature control for PCR using two eTEC thermoelectric modules that behave as microscopic Peltier heat pumps. Figure 4 shows example thermal profiles of the fluid in the well (“Water”) and the temperature of the sample holder (“Cu Cup”) that indicates how the TEC overdrives temperature to produce the desired thermal profile in the sample.
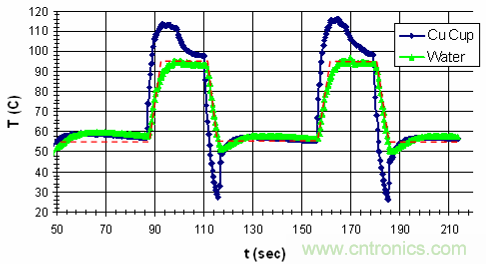
Figure 5: Transient temperature measurement using thin-films in PCR thermal cycling
Implementation of eTECs in PCR amplification provides the following advantages:
Shorter throughput times for DNA amplification
Fast temperature transition (10ºC per second)
Small mass of thermal cycler
Higher density of wells per machine
Physical size of conventional TEC prevents this versus the micro-size of eTECs
Precise temperature control
Ultra-fast response time
Uniquely high power pumping capability
[page]
Thin-Film Thermoelectrics Integration (Faster Cycles, Higher Throughput)
Thermal cycle times in PCR thermal cyclers are determined both by the dwell times during the denaturation, annealing, and extension phases and the thermal transition time between these phases. Thermal cycle time is minimized and throughput maximized by minimizing the transition time between the phases. Conventional PCR systems use large individual sample volumes (e.g.; 100 µL) and temperature transitions at 1 - 5 °C/s. However, while most PCR protocols are performed at the 25 µL to 50 µL scale, sample volume as low as 5 µL have also been shown to be successful.
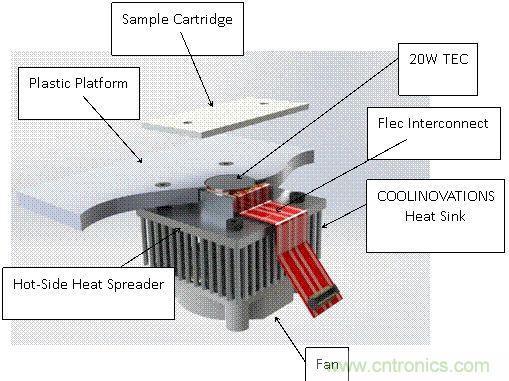
Figure 6: Typical structure of a PCR thermal cycler using thin-film thermoelectric devices.
A typical PCR thermal cycler using thin-film thermoelectric devices is shown in Figure 5. The thermal subsystem consists of a sample cartridge holder, support platform, thin-film thermoelectric module with integrated heat spreader interface, and heat sink (shown with fan). This subsystem is designed to provide rapid thermal cycling for single well or lab-on-chip designs. In conventional PCR systems intended for laboratory usage, multiple samples (e.g., 96 or more) are cycled simultaneously using a single large heat spreader and bulk thermoelectric device. Current market shifts towards doctor''''''''''''''''''''''''''''''''s office or patient side usage systems that handle 1 - 4 samples at a time are leading to a need for smaller and more rapid thermal cyclers.
The temperature transition rates (?C/s) between phases can be increased by the following:
minimizing the thermal capacity of the load (all components that must be heated and cooled)
minimizing the volume of the sample
minimizing the thermal resistance (conversely increase of thermal conductance) of the heat transfer structure on the sample side of the thermoelectric module.
optimizing the sample geometry to maximize the area for heat transfer into the sample cartridge and sample and to minimize the heat transmission distance in the sample. This can be achieved by integrating the sample side heat spreader into the thermoelectric module itself and matching its area with that of the sample area of the cartridge.
The high heat pumping capacity per unit area (typically measured in watts/cm2) of the thin-film modules, along with their inherent rapid response, enables extremely rapid temperature transitions in the sample. For optimized designs, temperature transition rates in the range of 20°C/s to 30°C/s are feasible for currently used sample volumes. For smaller sample volumes, even faster temperature transitions rates are possible.
A thermal cycler for a 50 µL sample volume was designed using a standard heat sink and fan with the sample chamber diameter of 16 mm and height of 0.25 mm. Typically used materials were assumed for the sample cartridge and film seal for the cycler shown in Figure 5. The thin-film thermoelectric module was optimized for the selected heat sink to minimize the thermal transition times. The modeled thermal response of the system achieves the 70°C to 95°C transition in the sample in 1 second and 95°C to 50°C transition in 2 seconds. The simulated temperatures of the sample (red line), sample side spreader (blue line), and heat sink (green line), are shown in Figure 6.
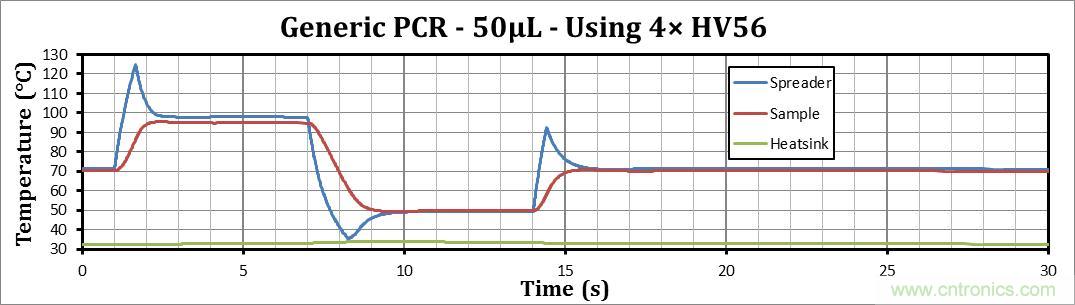
[page]
Electrical Considerations
The thin-film thermoelectric assembly as referenced in the PCR application above contains four eTEC HV56 modules connected electrically in series. The optimal drive current for these modules in a PCR application is likely to be in the -0.9A to 0.6A range. The range is achieved by applying negative (-) voltage to the assembly, which puts the eTEC into heating mode to achieve the 95C rise temperature, and then switching the polarity to put the eTEC into cooling mode to drive the device to the desired hold temperature.
Several off-the-shelf thermoelectric cooler controllers or temperature controllers are available to drive and control Nextreme eTEC modules. These controllers use current sensing to control the eTEC current. The devices rely on an external NTC thermistor to sense the load temperature and provide a control loop to adjust the eTEC current and maintain a desired load temperature.
Nextreme''''''''''''''''''''''''''''''''s eTEC module assemblies can easily be connected electrically in series or parallel configurations to match system voltages and drive current requirements.
Reliability of Thin-Film Thermoelectric Technology in PCR Thermal Cycling
Nextreme has conducted rigorous reliability tests on the HV-family of thermoelectric modules. The devices have surpassed baseline test in mechanical shock, thermal storage and power cycling. In all cases, the results strongly indicate HV modules are reliable in use in PCR thermal cycling applications.
Non-Powered Environmental Stress
High-temperature storage provides a good indicator of long-term reliability, particularly as it relates to diffusion-based failure modes and interface stability. The requirements for this test were 85?C for 2000 hours. To further stress the module and accelerate temperature dependent failure mechanisms, Nextreme subjects thermoelectric modules to temperatures well above maximum operating conditions (typically 150?C). Figure 7 shows the results of 150?C storage with read-points on the AC resistance at 254, 432, 1190, 1526 and 2534 hours. The AC resistance varied less than 1% from the starting resistance in all cases. This indicates there are no temperature driven changes in the thermoelectric material, contacts or interconnects under these conditions and the modules are stable.
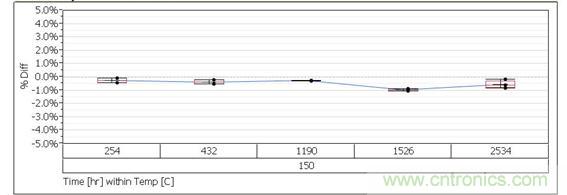
[page]
Powered Environmental Stress
Power cycling provides one of the most useful thermoelectric reliability tests as it simulates extreme usage by inducing large current pulses that simultaneously induce large thermal gradients in the device. These gradients produce expansion and contraction of the module that can lead to fatigue failures. Conducting power cycling at elevated temperatures can also induce diffusion-based failures. Figure 8 shows power cycling data of three HV14 modules at 90% of Imax for 100,000 cycles. In this test, the cycle period was 5 seconds on and 5 seconds off. This rapid cycling is enabled by the fast response time of the thin-film module and enables more rapid accumulation of data. It also results in a rapid change in the internal stress of the devices that contributes to accelerated aging and fatigue. The ?T of the module was monitored throughout the testing and exhibited less than a 4% change over the entire 100,000 cycle test.
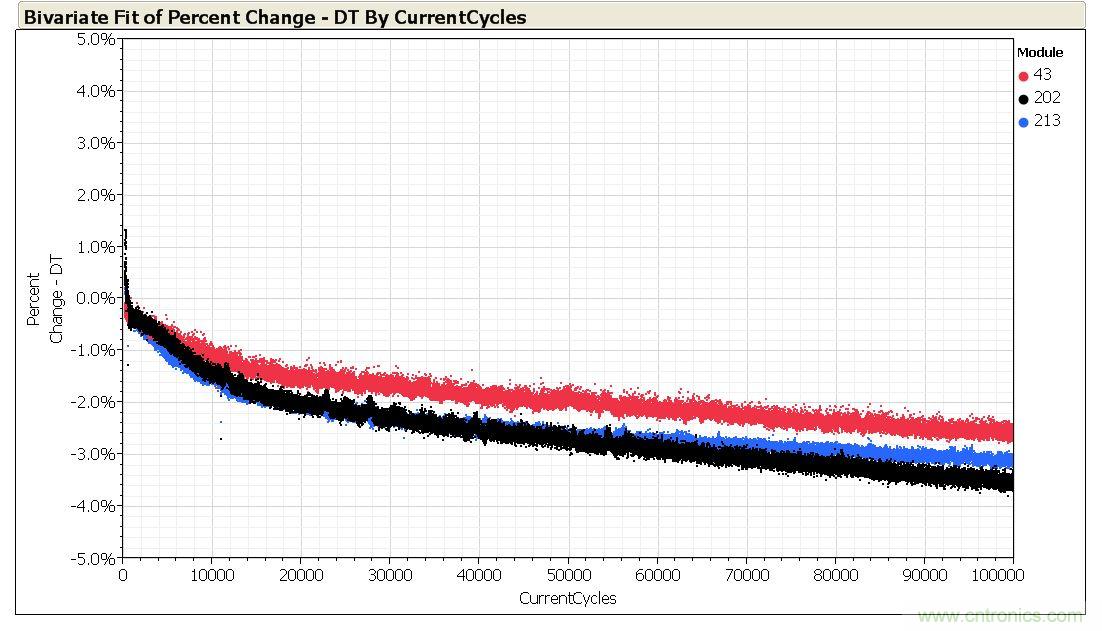
Figure 9: Change in module .T over 100,000 cycles at 90% of Imax.